Product Releases
July 9, 2025
Kong AI Gateway 3.11: Reduce Token Spend, Unlock Multimodal Innovation
Today, I'm excited to announce one of our largest Kong AI Gateway releases (3.11), which ships with several new…

Marco Palladino
CTO and Co-Founder
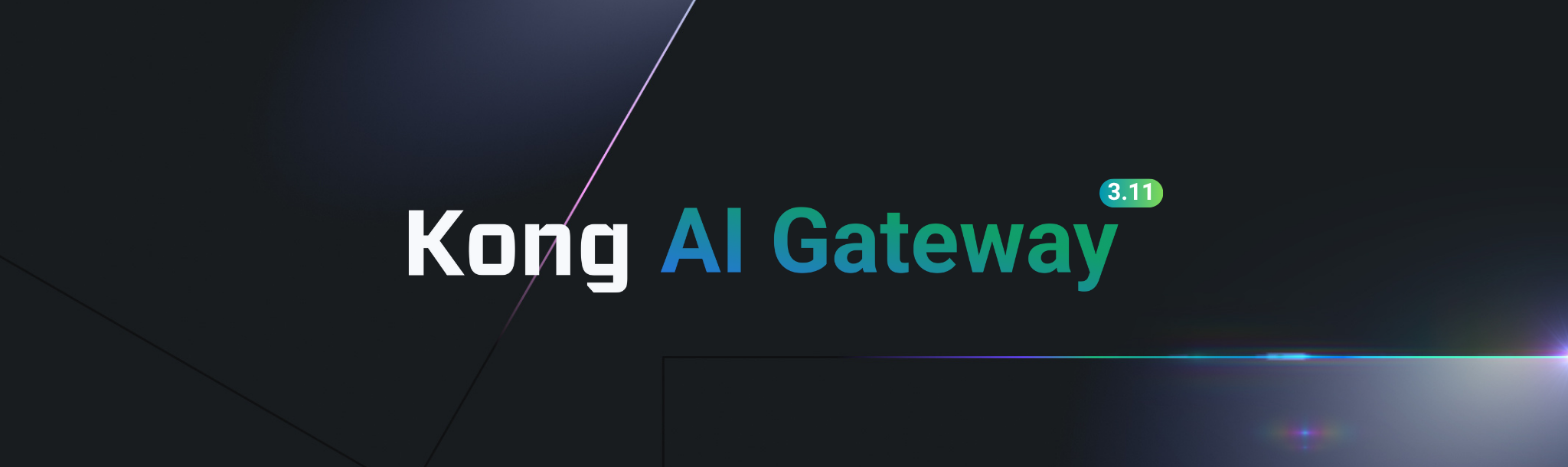
Talk tech with tips and tutorials from builders at Kong and the Kong Community.
Get insights from leaders changing business through API innovation.
Explore foundational guides unlocking key industry concepts and technologies.
Read up on what’s new and what's next in Kong’s latest product releases.